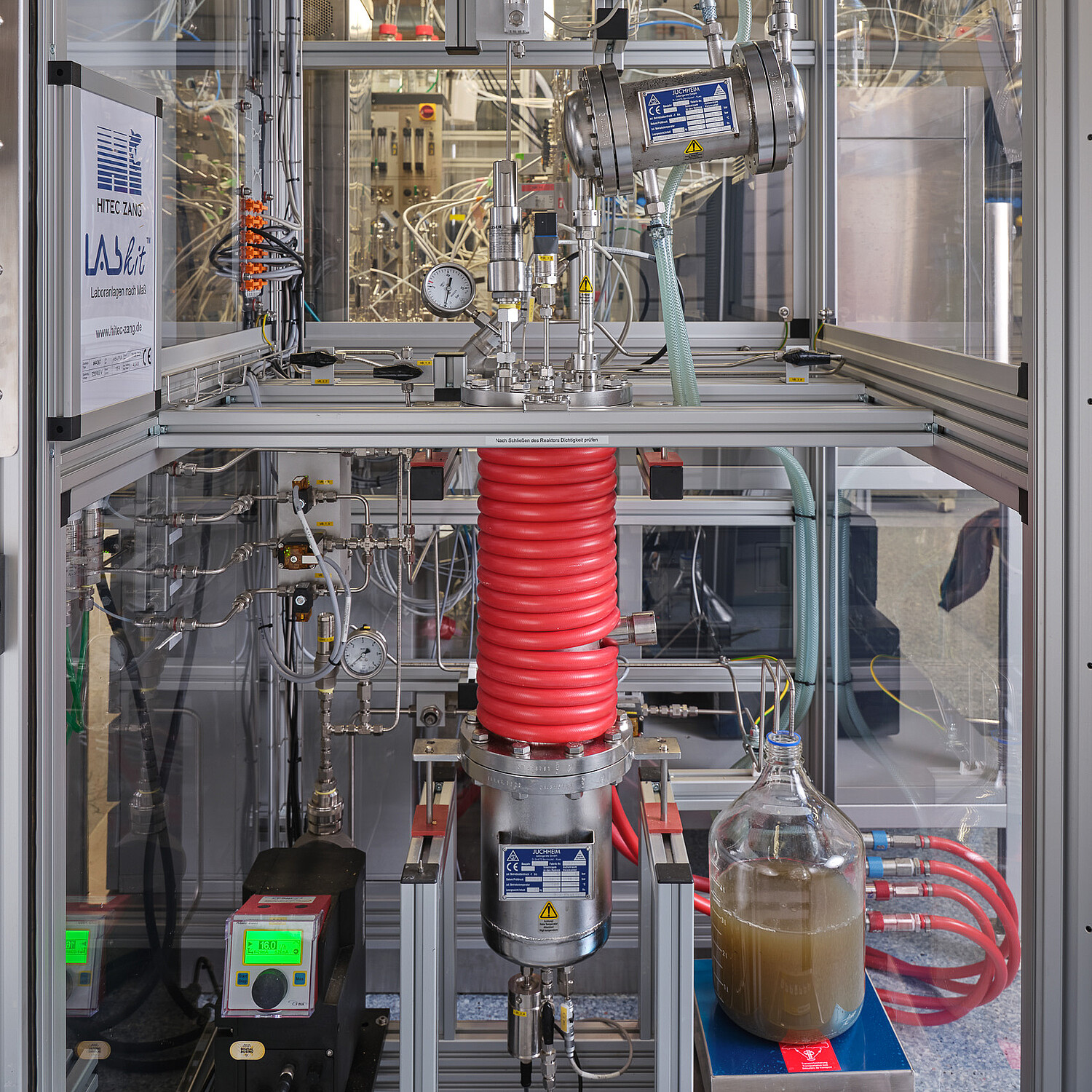
Storage technologies: how surplus power can be used sensibly
Surplus power can be stored temporarily in batteries or used to generate gas. In this way, fluctuations in production can be balanced out in an efficient and environmentally friendly manner, as demonstrated by new approaches used by ZHAW researchers. There are still some challenges to be overcome, however.
While the sun shines at its strongest at midday, power consumption peaks in the evening. This is set to pose a problem for the future energy supply, in which solar cells are to play a key role: Storage systems are required to ensure that the power is available at the right time.
Batteries represent an obvious solution here. However, conventional batteries have disadvantages, including the fact that they contain toxic heavy metals (cadmium, lead) as well as materials whose extraction is harmful to the environment or whose availability can fluctuate (lithium). Liquid batteries, which are also referred to as redox flow batteries, represent a new approach. Instead of solid matter, they contain liquids (electrolytes) that circulate in separate circuits. The battery's energy is found in the electrolytes, which are converted to a different oxidation state during charging and discharging. In the process, ions flow through a membrane that separates the two circuits.
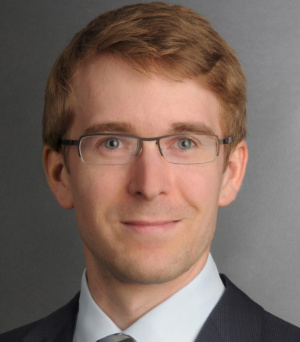
“The aim of the project is to create a simulation platform to search for organic molecules that are suitable for redox flow batteries.”
Various models of redox flow batteries are conceivable and development is in full swing. One promising idea is to use organic substances as a basis for the electrolytes. This approach is also being pursued by the Electrochemical Cells & Energy Systems research group, which is based at the ZHAW’s Institute of Computational Physics in Winterthur. It is involved in the EU Horizon project SONAR, which will run until 2024. “The aim of the project is to create a simulation platform to search for organic molecules that are suitable for redox flow batteries,” says Roman Schärer, a research associate within the research group. “There is an enormous range of potentially suitable molecules and this potential is a long way from being exhausted.”
Long-life batteries
The research work is not only being conducted in the laboratory, but also on screens. In fact, almost the entire EU project is based on modelling at different scales and computer simulations. For example, the behaviour of electrolytes is being simulated at a molecular level, the ideal geometry of the battery stacks is being sought and the long-term profitability of entire plants is already being forecast. After all, the organic molecules they are seeking not only have to ensure reliable power storage, but also guarantee competitive operating costs. A decisive factor for this is longevity. Redox flow batteries are said to have a service life of up to 20 years with a very low level of material degradation.
Less dependence
However, the security of supply also plays a role. “We are currently experiencing what it means to be dependent on individual energy sources on an overly one-sided basis,” says Jürgen Schumacher, head of the Winterthur-based research group. “The EU project is therefore looking for solutions that work within the EU.” The chosen approach with the organic molecules has the potential to make Europe independent of sensitive imports, in this area at least.
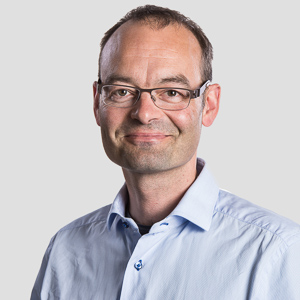
“The materials used should be as recyclable as possible and must exhibit a low hazard potential.”
Environmental friendliness is a further important criterion. “The materials used should be as recyclable as possible,” says Jürgen Schumacher. “And they must exhibit a low hazard potential.” This is not only with respect to their toxicity: As liquid batteries have a smaller energy density than lithium-ion batteries, they are less flammable. At the same time, this low energy density is a disadvantage, as it implies an increased space requirement. However, if such a battery were to be located in a residential area, this downside would likely be accepted in favour of improved safety.
Battery containers for groups of buildings
Redox flow batteries are often installed in commercially available containers. In the future, it would be conceivable for groups of buildings, for example, to each have their own battery container powered by wind and solar energy. “This would not only increase energy security, but also democratise and decentralise the power supply to a certain extent,” says Jürgen Schumacher. He adds, however, that for certain applications there is still a need for energy carriers with a high energy density, such as hydrogen.
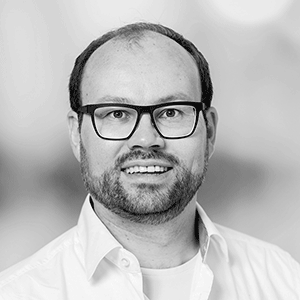
“Power-to-gas is the central coupling element between power and gas infrastructure. This is why it will become the key technology in the energy transition.”
While batteries can help to balance short-term fluctuations in the power grid, the problem of seasonal balancing remains. Solar and, to some extent, hydroelectric power are primarily generated in the summer, while consumption reaches its highest levels during the winter. It is questionable whether Switzerland will also be able to cover its winter shortfall with imports in the future. It would be helpful if the excess power generated during the summer months could be transferred to the winter. Batteries are of little help here: The number of units required would be far too high.
Converting power to gas
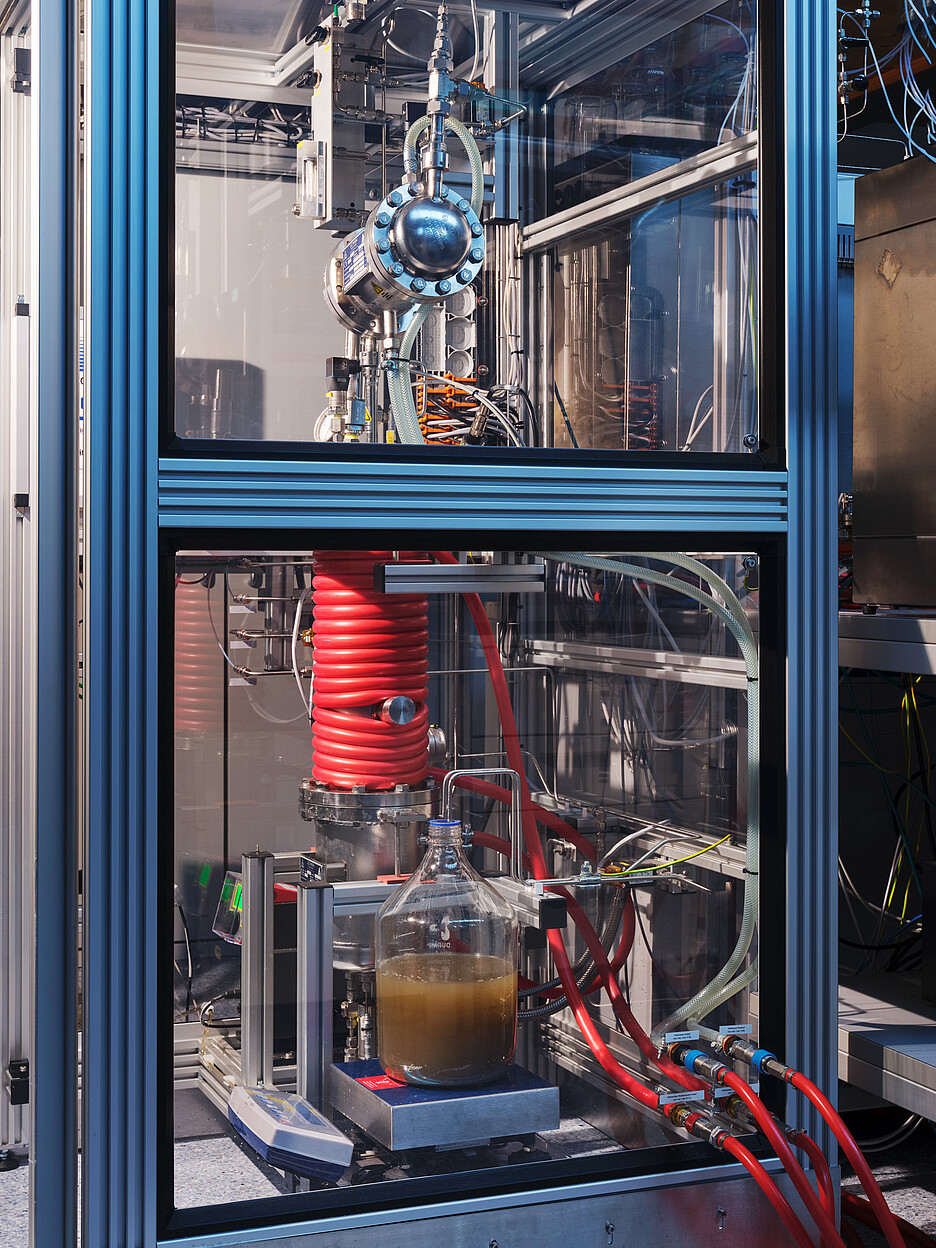
One solution is to use power-to-gas technology. The idea behind this approach is to use the surplus power to generate gas, which is easier to store than electricity. The gas can either be consumed directly or converted back into power during the winter when demand is at its highest. “Power-to-gas is the central coupling element between power and gas infrastructure,” says Hans-Joachim Nägele, Head of the Section of Environmental Biotechnology and Bioenergy at the ZHAW’s Institute of Chemistry and Biotechnology based in Wädenswil. “This is why it will become the key technology in the energy transition.”
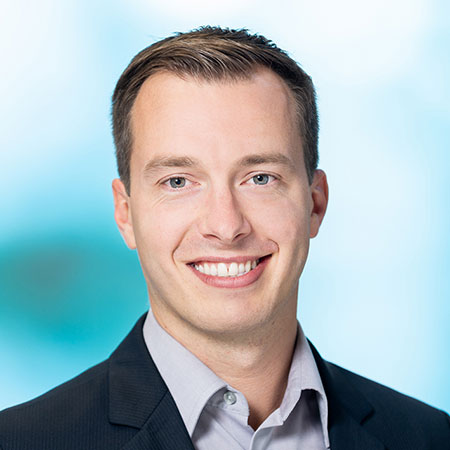
“We are pursuing the approach of converting hydrogen together with CO2 into methane, the main component of natural gas.”
The first step of the process comprises electrolysis and sees electricity being used to break down water into its components of hydrogen and oxygen. While the hydrogen could now be stored, it is volatile and the corresponding infrastructure is still lacking. “We are pursuing the approach of converting hydrogen together with CO2 into methane, the main component of natural gas”, says Nägele’s colleague Wolfgang Merkle. The best thing of all is that methane can be fed directly into the natural gas grid, meaning the required infrastructure is already in place. “And the grid also serves as a storage system,” says Merkle. “The pipelines and gas storage facilities house a quarter of the natural gas that Europe consumes in a year.” When the artificially produced methane is subsequently used to generate power, this is done in a climate-neutral manner, as it is only the CO2 that was previously extracted from the environment and used in the gas synthesis process that is released during combustion.
Environmentally friendly methane production
The production of methane needs to be optimised, however, and this is what the Wädenswil-based group is researching. The laboratory is still home to a small experimental reactor from the final phase of the now complete EU CarbonATE project in which the group was involved. This project focussed on a more environmentally friendly method of methane production that utilises biological rather than chemical processes. The process and the reactor are currently being investigated further.
Help from microorganisms
The work in the reactor is performed by archaea, which are bacteria-like single-celled organisms that form their own domain in the tree of life. They are able to produce methane from carbon dioxide and hydrogen. “This is less complex from a technical standpoint and therefore cheaper than conventional methods,” says Hans-Joachim Nägele. The biological method also requires little energy: There is no need for any heating, as the required heat of just below 45 degrees Celsius is generated without the need for any further input during the methane synthesis process. Chemical methanation, on the other hand, is energy-intensive – it requires pressure of up to 200 bar and a temperature of 300 to 500 degrees Celsius. It is also more sensitive to impurities, such as hydrogen sulphide, which makes the use of waste gas as a source of CO2 more laborious. However, the chemical process also works much faster than its biological counterpart.
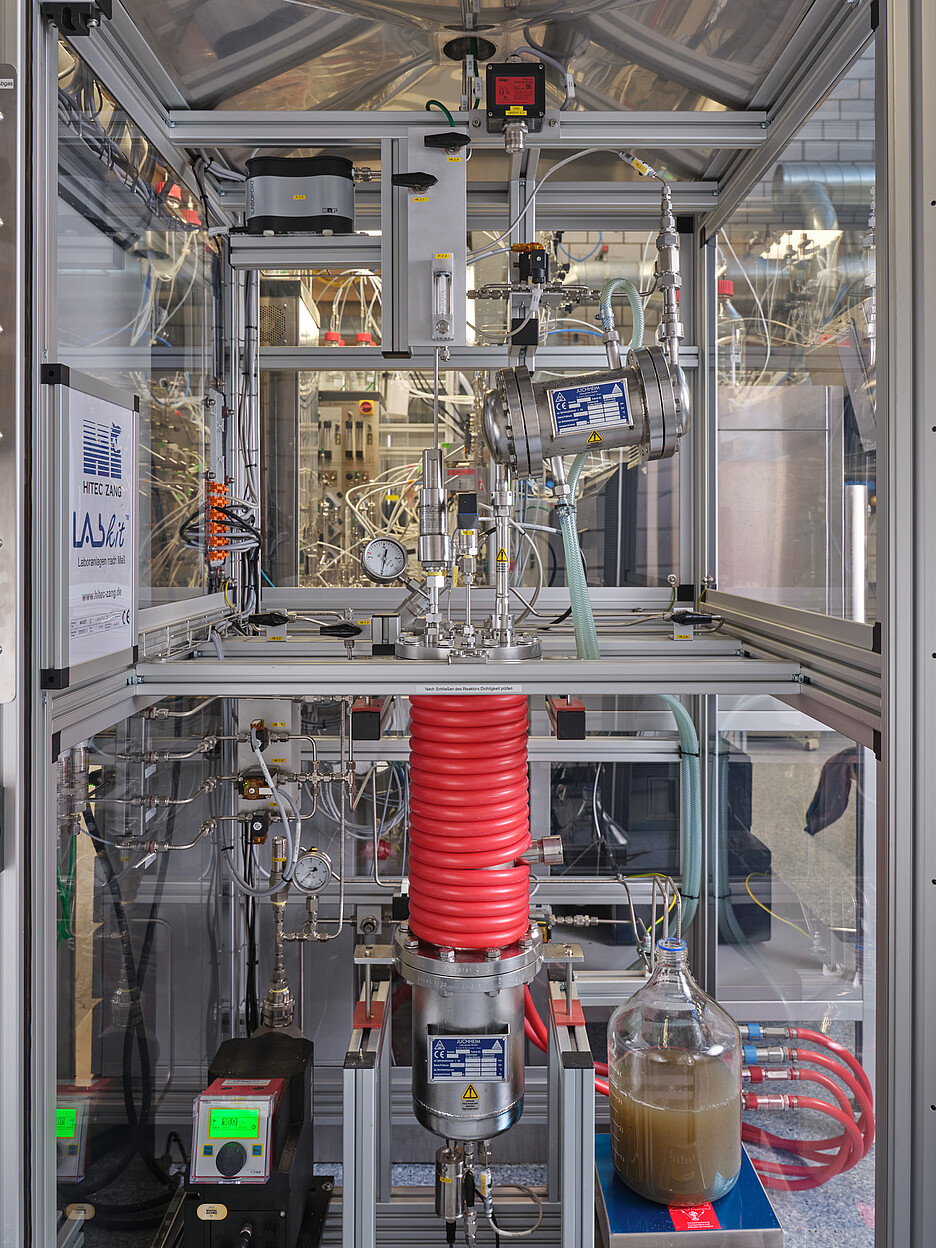
At farms, cement plants and sewage works
The question remains as to where such power-to-gas plants will one day be located. The Wädenswil-based researchers are initially focusing on farms: “In Switzerland, there is still a great deal of untapped potential, namely in the form of slurry and solid manure,” says Merkle. This could be used in biogas plants, generating CO2 and methane in the process. Merkle adds that the CO2 could be used in a second step to produce further methane, i.e. with power-to-gas plants. However, larger reactors that would really make a difference in combating the winter electricity shortfall would have to be built in places where major sources of CO2 are available, for example at sewage works, waste incineration plants, cement works and larger biogas plants.
70 percent of output energy is lost
There remains a catch: Converting solar power into gas and then back into power at the end is a complex undertaking – the efficiency of the whole process stands at between 30 to 40 percent, which means that some 60 to 70 percent of the output energy is lost. “It is for this reason that power-to-gas is only worthwhile if we really have surplus power,” says Merkle. “But if we do, it makes sense. Otherwise, this power would simply be lost.”
0 Comments
Be the First to Comment!